Scientists explore nature’s promise in combating plastic waste
May 8, 2024
- Since 1950, humanity has produced more than 8.3 billion metric tons of plastic. Most has ended up in landfills or the environment. Now, scientists are working on biological solutions to address the plastic pollution crisis at every stage of the material’s life cycle.
- Innovative new filters built from naturally occurring ingredients can capture micro- and nanoplastics in all their diverse forms. These filters could remove plastic contamination from drinking water, and prevent microplastic pollution in industrial and domestic wastewater from reaching rivers and oceans.
- Plastic-degrading enzymes, isolated from microbes and insects and engineered for efficiency and performance in industrial conditions, can break plastics down at the molecular level and even be used to turn plastic waste into new useful chemicals.
- Biological solutions are being developed for a range of pollutants, not just plastics. But this technological research is still young. Crucially, we must not allow solutions for existing pollutants to make us complacent about the impact of new chemicals on the environment, or we could risk making the same mistakes again.
Plastic is a remarkably versatile and durable material, which has made it indispensable in almost every area of modern life. But these same properties, amplified by our “take-make-waste” linear economy, have created a brewing environmental catastrophe.
It’s hard to grasp the gigantic scale of our global plastic waste problem. Since 1950, humanity has produced more than 8.3 billion metric tons of plastic. Most has ended up in landfills or the environment, where it is harmful to wildlife, ecosystem functioning and human health.
Once in the environment, plastic slowly breaks down into smaller and smaller fragments, known as micro- and nanoplastics. But it can take hundreds or even thousands of years for plastic, which itself contains thousands of chemicals, to degrade into its basic natural components. That’s because plastics are made up of repeating units of tightly bonded carbon-rich molecules, which makes them very difficult to break down.
But nature brings cause for hope: scientists are working on a range of biological solutions to address the plastic pollution crisis at every stage of the material’s life cycle, from capturing waste before it enters the environment, to upcycling plastics into new, useful products.

Nature-based filters: Microplastics come to a sticky end
Micro- and nanoplastic pollution is a fast-growing global issue, with few practical solutions. While larger pieces of plastic waste can be laboriously collected, these tiny fragments represent a much bigger challenge to clean up.
“Nano- or microplastics are really challenging [to capture] because they each have a different size, different chemical composition, different hydrophobicity. So, there’s no single material that can remove the wide spectrum of nano- or microplastics,” says Junling Guo, a materials scientist at Sichuan University in China.
But there’s hope: Guo led an international research team that developed a plant-based filter that can capture micro- and nanoplastics suspended in water, which they named bioCap.
The filter is composed of a sawdust base laced with plant tannins, or polyphenols — chemicals produced by plants to repel hungry herbivores, and also responsible for that dry sensation we get after drinking tea or red wine. This mouth-puckering effect is what makes tannins ideal for capturing microplastics.
“One of the most interesting properties of polyphenols is that they are sticky molecules,” Guo explains. Recognizing this trait, he realized it might be possible to “use the polyphenol as a nano glue to capture the microplastics regardless of their chemical compositions, or any other properties.”
The chemical characteristics of polyphenols allow bioCap to trap plastic particles of all types. “Polyphenols can form multiple molecular interactions with different types of nano- or microplastics,” says Guo. In laboratory tests, the bioCap filter captured between 95 and 99% of plastic particles in a water sample.
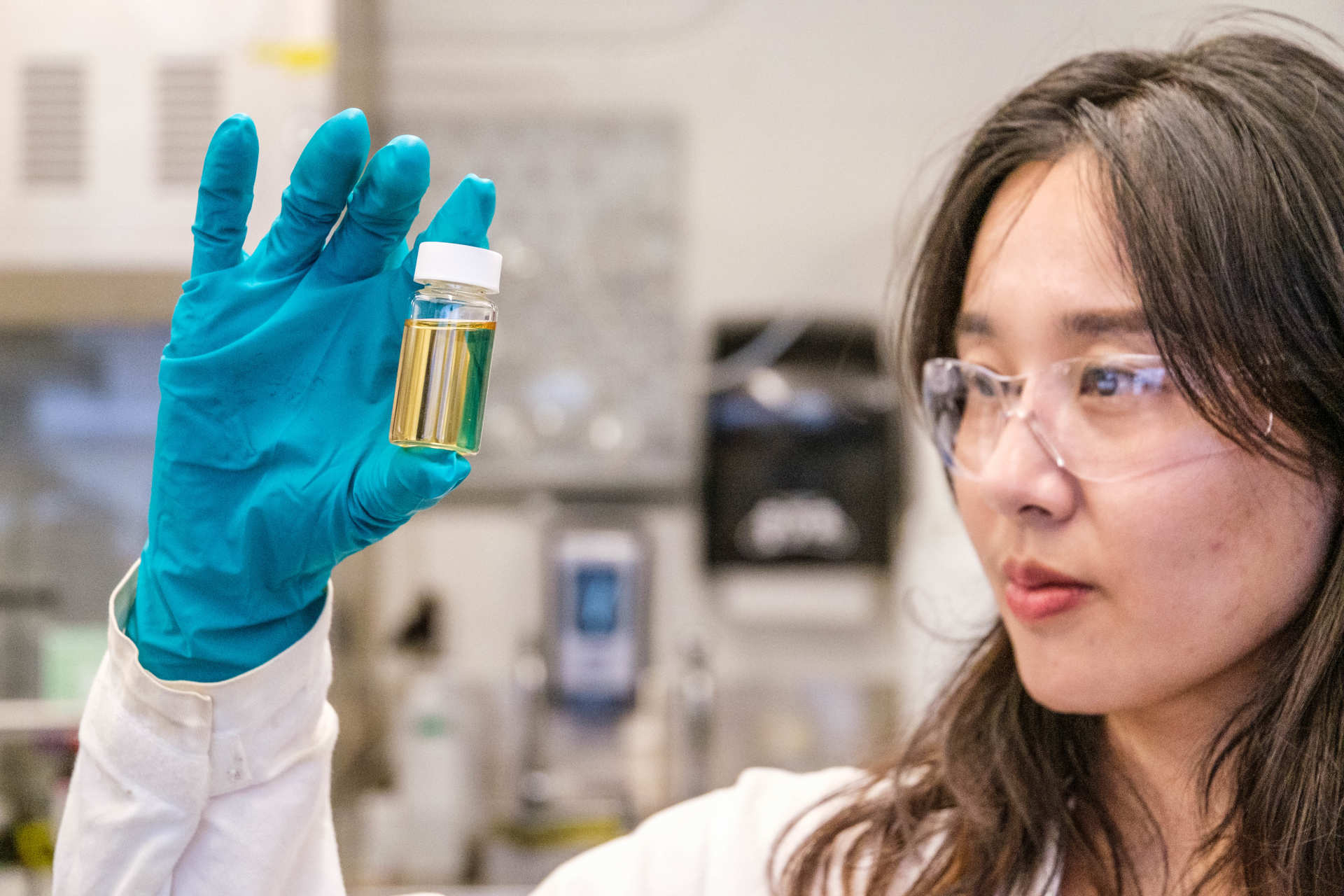
A major advantage of this approach is that it’s natural: All components in a bioCap filter are naturally occurring and fully biodegradable. And tannic acid is already an FDA-approved food ingredient. “It’s biocompatible and relatively low cost,” Guo says.
Nature-based filter technology is a growing field of research. In 2022, scientists in Finland reported initial tests of a cellulose nanofiber filter made from wood pulp that can capture polystyrene micro- and nanoplastic particles suspended in water.
And scientists in Hong Kong have demonstrated that microscopic films of bacteria, known as biofilms, also have sticky properties that can capture microplastics and then release them on demand, ready for recycling or upcycling.
Filters like these could be a game-changer for removing microplastic contamination in drinking water, and prevent pollution from industrial and domestic wastewater reaching rivers and oceans. But Guo acknowledges such technologies aren’t a complete solution to plastic pollution: “We use a polyphenol to capture the micro- and nanoplastics, but they are still there,” needing to be disposed of somehow.
Guo’s team is currently collaborating with other scientists who are developing plastic-degrading enzymes, and hope to eventually incorporate these into the bioCap filter, creating a “complete loop to eliminate micro- and nanoplastics,” he says.

Molecular breakdown
Most plastic waste today is incinerated or dumped in landfills; less than 10% is recycled into new products. On top of that, current recycling methods tend to produce plastic of lower quality, so there is an upper limit on how many times a piece of plastic can be recycled before it reaches its end of life.
In truth, as much as 95% of the material value of plastic is lost after its first use. The challenge is to find a circular economy solution to reuse plastic again and again.
Scientists have been searching for this holy grail — a biological solution to break down plastic at a chemical level — for decades. But interest has surged since 2016, when a research team at Japan’s Kyoto Institute of Technology, led by Kohei Oda, successfully isolated a bacterial enzyme capable of breaking down polyethylene terephthalate (PET), a very common plastic used in textiles, plastic bottles, and other packaging, with around 70 million metric tons manufactured globally each year.
A variety of plastic-degrading enzymes have since been isolated from bacteria and fungi, with researchers around the world engineering and tailoring them for industrial purposes. These enzymes could be synthesized chemically and put straight to use, or microbes like E. coli could be engineered to produce them for us.
The first commercial plastic-degrading enzymes may not be far off. French company Carbios is pioneering a PET-degrading enzyme in the commercial market. The company has engineered the enzyme (initially isolated from bacteria in compost) to improve efficiency. The chemical reaction breaks PET down into its main constituents — terephthalic acid and ethylene glycol — which can then be used to make new plastics without the loss of material value associated with traditional recycling approaches.
In 2022, Carbios announced plans to open the world’s first industrial-scale enzymatic PET biorecycling plant in Longlaville, France, a facility set to begin operations in 2025. The plant will be able to process 50,000 metric tons of post-consumer PET waste annually, the firm says — that’s around 2 billion PET plastic bottles.
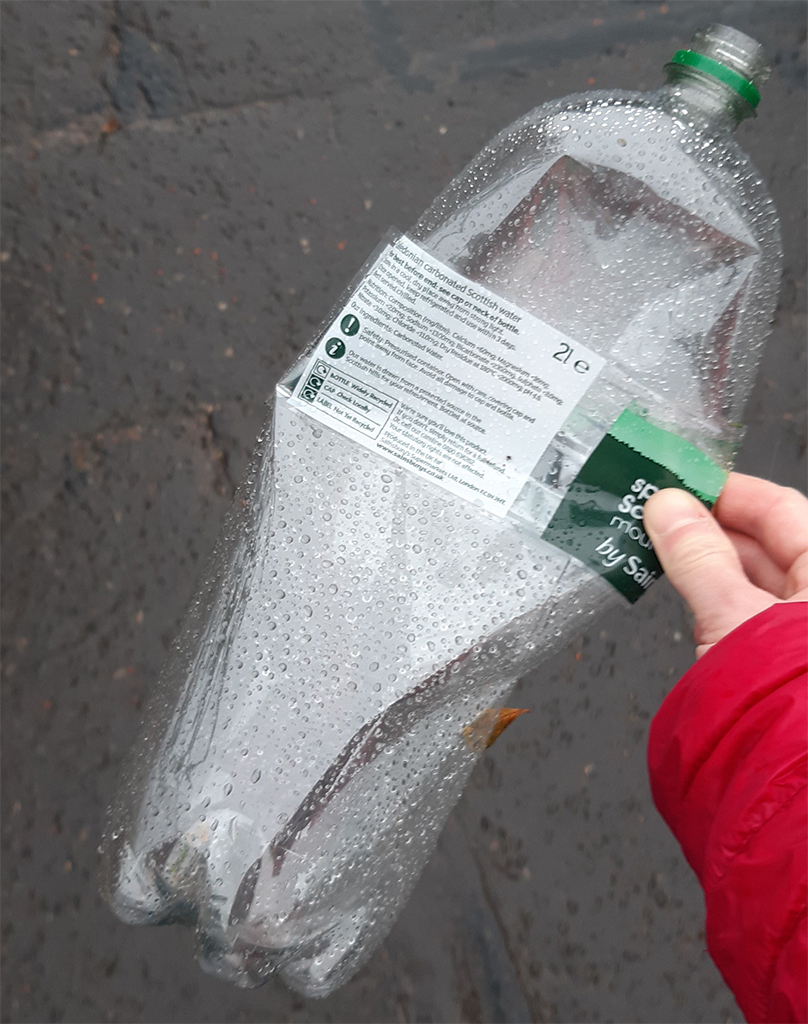
Plastic-munching caterpillars
Biological breakdown of other types of plastic have proved more elusive, but progress is being made. In 2017, Federica Bertocchini, a molecular biologist at the Spanish National Research Council in Madrid, discovered that caterpillars of the wax moth species (Galleria mellonella) can break down polyethylene (PE), a common plastic used in packaging. Since then, Bertocchini and her colleagues have worked to understand how the caterpillars achieve this feat, and to isolate the enzyme or enzymes responsible.
Bertocchini’s team has now tracked the plastic-degrading effect to the caterpillar’s saliva. The enzymes found there produce a variety of chemical products that have useful applications, for example in the manufacture of nylon and cosmetics.
In 2022, Bertocchini co-founded Plasticentropy, a biotechnology company that hopes to bring these plastic-degrading enzymes to market. The scale of the plastic waste problem is formidable, but Bertocchini is optimistic and convinced that wax worm enzymes can be part of the plastic waste solution when scaled up.
“There are mountains [of plastic waste,] so if I said we could degrade all the plastics in the world with these enzymes right now, it wouldn’t be believable,” notes Bertocchini. Instead, “we can start at a small scale,” and slowly increase capacity. Her aim is to optimize the degradation process catalyzed by these enzymes, so they can be used within waste management facilities, or even people’s homes.
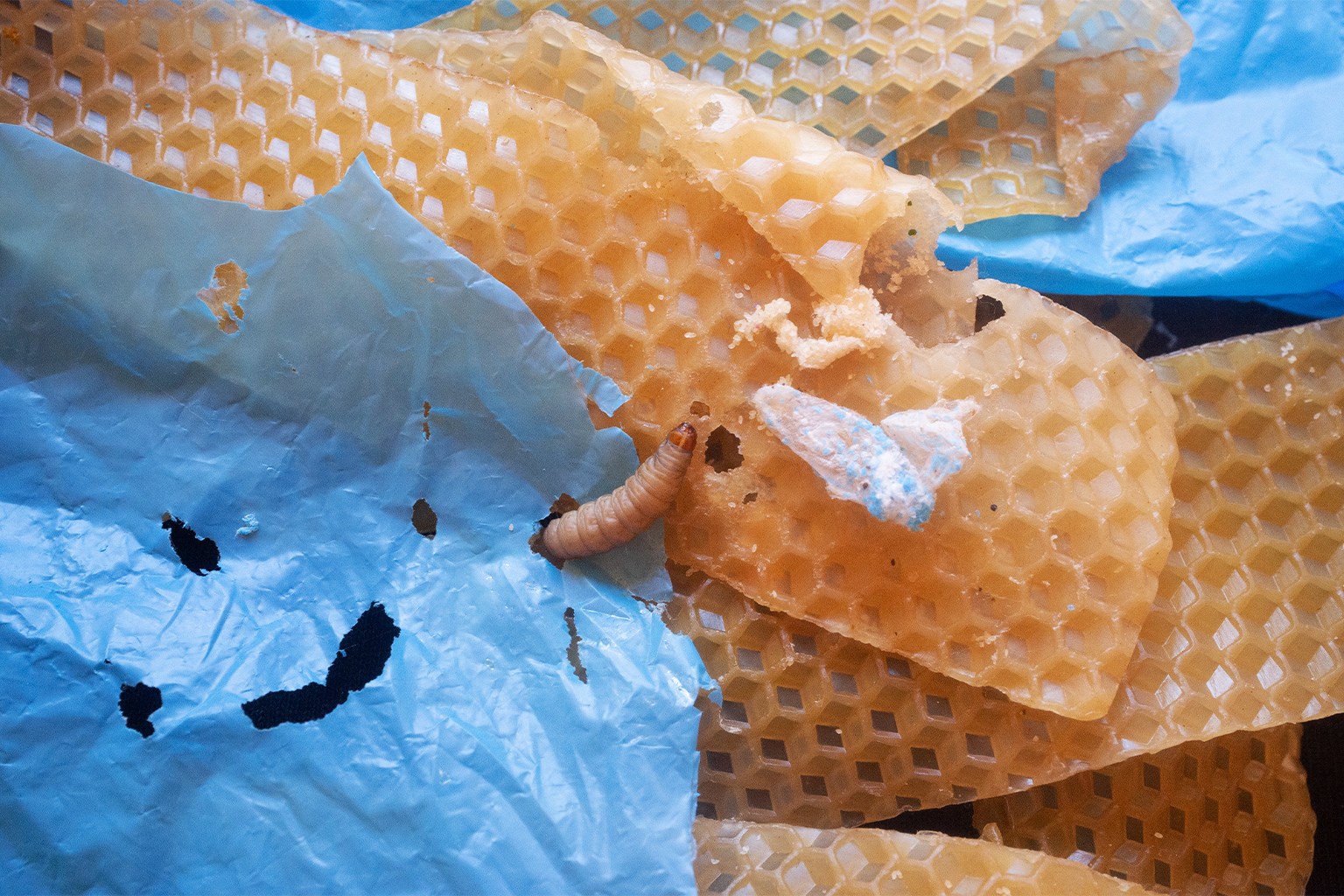
A tasty solution
Biological solutions able to break down plastics into their chemical components are only part of the story, though. “We need to move away from just thinking about how we depolymerize the plastic and [move toward thinking about] what we do with the plastic after that,” says Joanna Sadler, a biotechnology research fellow at Scotland’s University of Edinburgh.
Currently, “the chemical pathways we can access using … the existing toolbox [of enzymes] is a little bit limited,” she explains. This, in turn, limits the chemical products that can be generated from plastic waste.
Take PET degradation, for example: “We don’t necessarily want lots of terephthalic acid and ethylene glycol in the world. There’s a limit to how useful that is if you just stop there,” Sadler explains.
Sadler is developing microbes able to upcycle plastics into new, useful chemicals that could be part of a circular economy. For example, she and her colleagues have engineered E. coli to convert PET into vanillin, the chemical responsible for the taste and smell of vanilla, using a suite of six enzymes.
Vanillin is an important and versatile chemical, used as a flavoring in the food industry, as a fragrance in the perfume industry, and as a bulk chemical in the pharmaceutical and agrichemical industries. Current global demand for vanillin stands at 35,000 metric tons annually, and the industry was valued at more than $600 million in 2022. Some vanillin is produced naturally by harvesting and processing vanilla pods, but much of the global supply is synthetic, derived from petroleum.
Sadler’s brainwave came when she realized vanillin is chemically related to terephthalic acid, a main product of PET degradation. That insight helped her engineer a sequence of enzymes able to turn plastic bottles into this sweet-smelling, very useful chemical.

Sadler hopes that these engineered microbes will become part of a circular upcycling process that will replace a percentage of synthetically produced vanillin. “We’re now working with industrial collaborators to see whether this is something that could be used at the industrial scale,” says Sadler.
However, the technology needs optimization. The vanillin quantities produced initially were “simply too low to be industrially viable and to be competitive with existing technologies,” she notes. A further challenge: vanillin is toxic to microbes like E. coli, so “if you start accumulating too much, you start killing the thing that’s trying to make it.”
Once these hurdles are overcome, “we will be able to deliver a vanillin product which is chemically identical to that currently derived either from oil or from natural sources,” says Sadler. “I see no reason why it can’t be put into food, if it undergoes rigorous safety tests,” she says. However, she believes the fastest route to market is likely via the fragrance or chemical industries.
At the same time her team develops upcycling microbes for existing plastics, Sadler hopes to “future proof the plastics industry,” by investigating ways to upcycle new bioplastics just coming onto the market. Although bioplastics are biodegradable by design, she believes we should “think more circularly about it and have some technologies [at hand able] to take that carbon that’s locked up in plastic, upcycle it, and put it back into the economy.”

Micro-sharing the plastic burden
While much research into the biological degradation of plastics has focused on enzymes, or suites of enzymes inside a single bacterial strain, some researchers are taking a more community-minded approach.
Ting Lu, a synthetic biologist at the University of Illinois Urbana-Champaign (UIUC), and his team are genetically engineering interacting microbial strains that, as a community, can upcycle PET plastic, using a technique known as microbial ecosystem engineering.
Microorganisms are “single-celled organisms, but they are highly social,” he explains. “They often interact together to form complex, multispecies communities.” By engineering multiple interacting strains, scientists can leverage the principle of division of labor to “achieve a common goal by dividing tasks into smaller subtasks among community members,” says Lu.
The microbial community Lu and his team have created consists of two genetically engineered strains of the Pseudomonas putida soil bacterium. “Each strain is specialized in degrading one of the compounds, but together as a community, these strains achieve a complete utilization of the PET hydrolysate,” Lu explains.
Division of labor has helped human societies flourish, and is beneficial to many insect societies as well, so why not apply it to one of humanity’s greatest challenges, asks Lu. Dividing the complex chemical tasks of plastic breakdown between different bacterial strains allows each strain to become a specialist in its subtask, and allows researchers to “fine-tune the system performance to enhance overall productivity.”
But the team didn’t stop there: “We introduced an additional metabolic pathway into the two bacterial strains, so they are able to convert the carbon released from plastic into valuable chemicals,” Lu says. The resulting synthetic microbial community converts PET into polyhydroxyalkanoate (PHA), a biodegradable polymer that could be used to produce bioplastics, and muconic acid, a chemical building block used by the agrichemical and pharmaceutical industries.
Lu’s team has tested its microbial consortium in 1-liter (34-ounce) mini bioreactors, but the scientists acknowledge there is still much research needed to prepare the technology for industrial use. “Scaling up is not trivial,” he says. But Lu is optimistic his lab’s system approach will be market-ready to apply at industrial scale “in the next five to 10 years.”
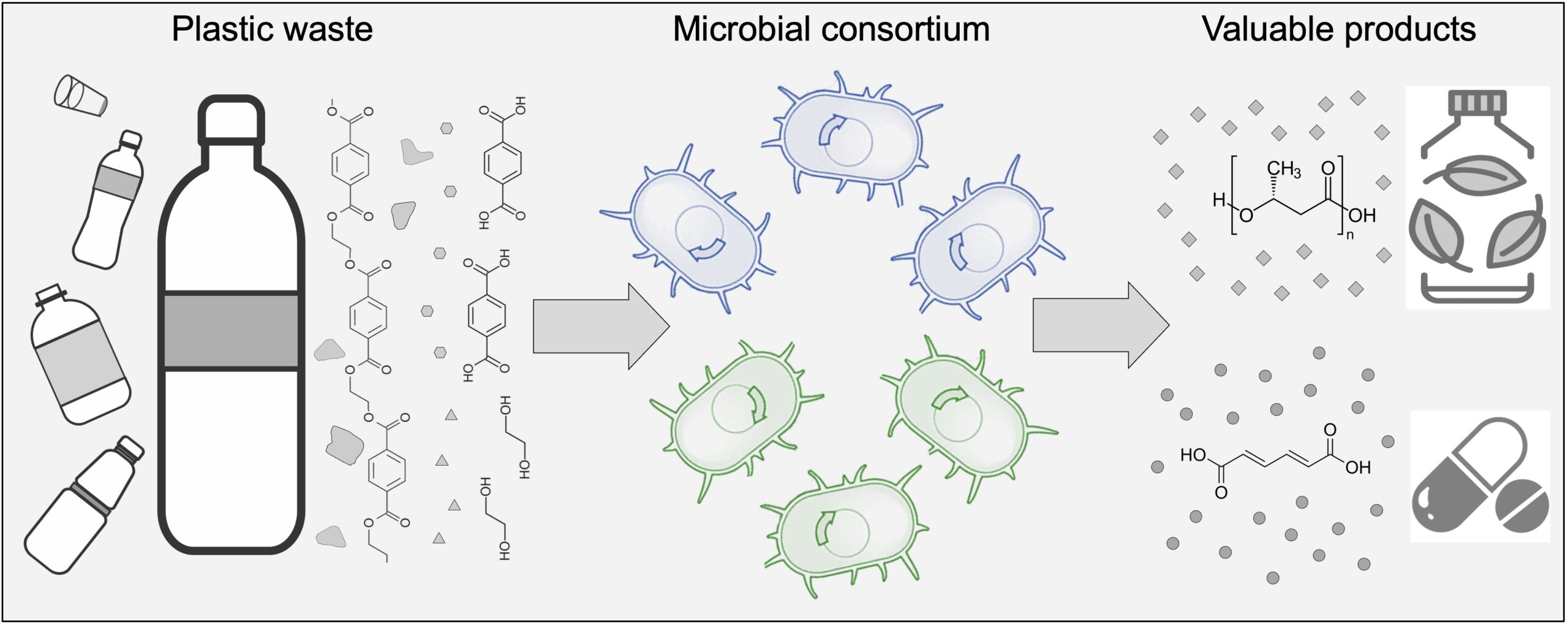
The long road ahead
While laboratory experiments have produced extremely promising results, challenges remain. To compete with traditional approaches, such as mechanical recycling or incineration, biological recycling and upcycling technologies must be fine-tuned to increase efficiency and maximize the quantity and quality of useful chemicals they produce.
But progress is being slowed by lack of funding. Environmentalists and scientists may be keen to find solutions to plastic waste, but “industry is not investing much,” Bertocchini says.
The future is even less certain for plastic cleanup technologies, which don’t yet yield any commercially valuable products. Although these cleanup methods may never be profitable, they’ll be crucial in the fight against microplastic pollution.
“The public and government have not yet fully acknowledged the significant harm posed by nano- or microplastics,” explains Guo. But “Given that the development and deployment of microplastic removal technologies are unlikely to be commercially profitable, it is crucial for governments to step in and provide the necessary policy support.”
Recycling and upcycling solutions face myriad other challenges as they move from the lab to the real world. First off, they must contend with many of the same problems faced by traditional recycling. Plastics come in a dizzying array of types, which each need to be sorted and processed separately. “The steps you need to put different materials through in order for them to be suitable for this kind of upcycling technology are very different depending on what that material starts out to be,” explains Sadler.
Plastics also commonly contain a complex mixture of additives, which can interfere with the chemistry needed to break down and recycle the plastic into new useful products.
And then there are the contaminants arising from the use of the plastics, such as food and hazardous chemicals, that can also disrupt the recycling or upcycling process.
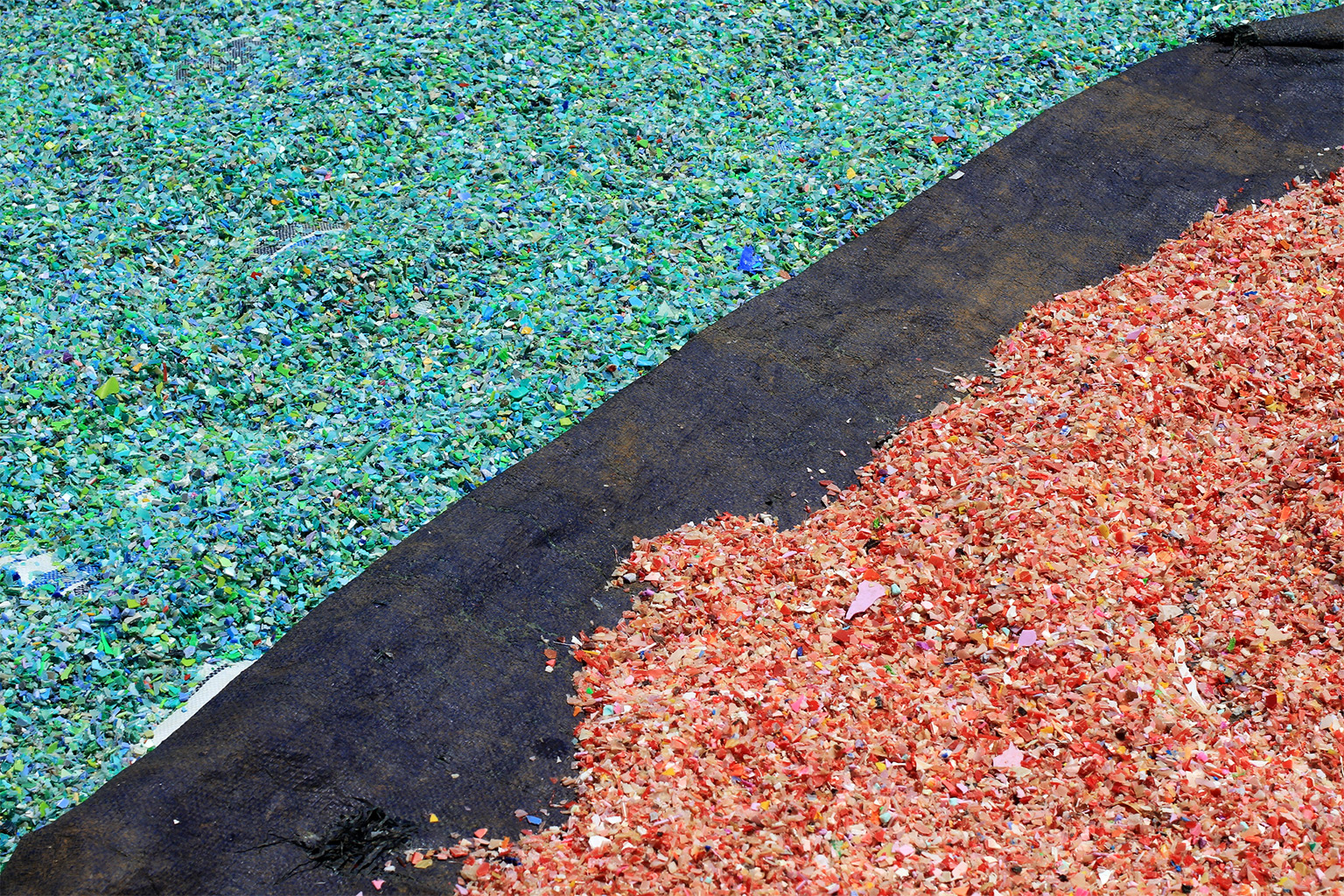
Fortunately for the environment and humanity, biological solutions may be able to tackle these challenges head-on. A recent laboratory study using engineered soil bacterium pioneered a novel method that broke down a mixture of common plastics into small molecules that were then converted into a useful chemical product.
Another problem shared with traditional recycling is the need for pretreatment. Plastic is so difficult to break down that most processes require pretreatment steps, usually performed at high temperatures and often requiring hazardous chemicals. “The most energy-intensive part of plastic degrading processes is the need to pretreat” the plastic, Sadler says.
Several researchers who spoke to Mongabay said they were working on biobased processes to eliminate these energy-intensive pretreatment steps.
Putting nature-based solutions to work
A key question, as these new technologies mature, is where and how they can most effectively be deployed. Experts agree that biological plastic recycling or upcycling solutions are best applied within dedicated bioreactors, situated at landfill sites or other waste-collecting locations — before waste enters the environment. To achieve this, scientists and technologists need to “interact with industries at the waste generation point … and intercept post-consumer plastic before it gets discarded,” says Sadler.
The alternative — releasing plastic-degrading enzymes or microbes directly into the environment to degrade plastics — comes with serious problems, ranging from environmental safety and ethical concerns, to the need to collect the useful chemical products of plastic breakdown for reuse once they have diffused into the wider world. Also, as microbes act on plastics, toxic additives and other pollutants bound to it would be released to the environment, posing health risks.
That’s why the release of genetically engineered microbes into the environment to clean up waste is not “a particularly useful or sensible way forward,” Sadler says.
But some researchers hope plastic degradation will take place in the environment one day. Lu says his vision is to “engineer microbes to break down the plastic in the landfill and then release carbon substitutes that could serve as fertilizers” for crops.
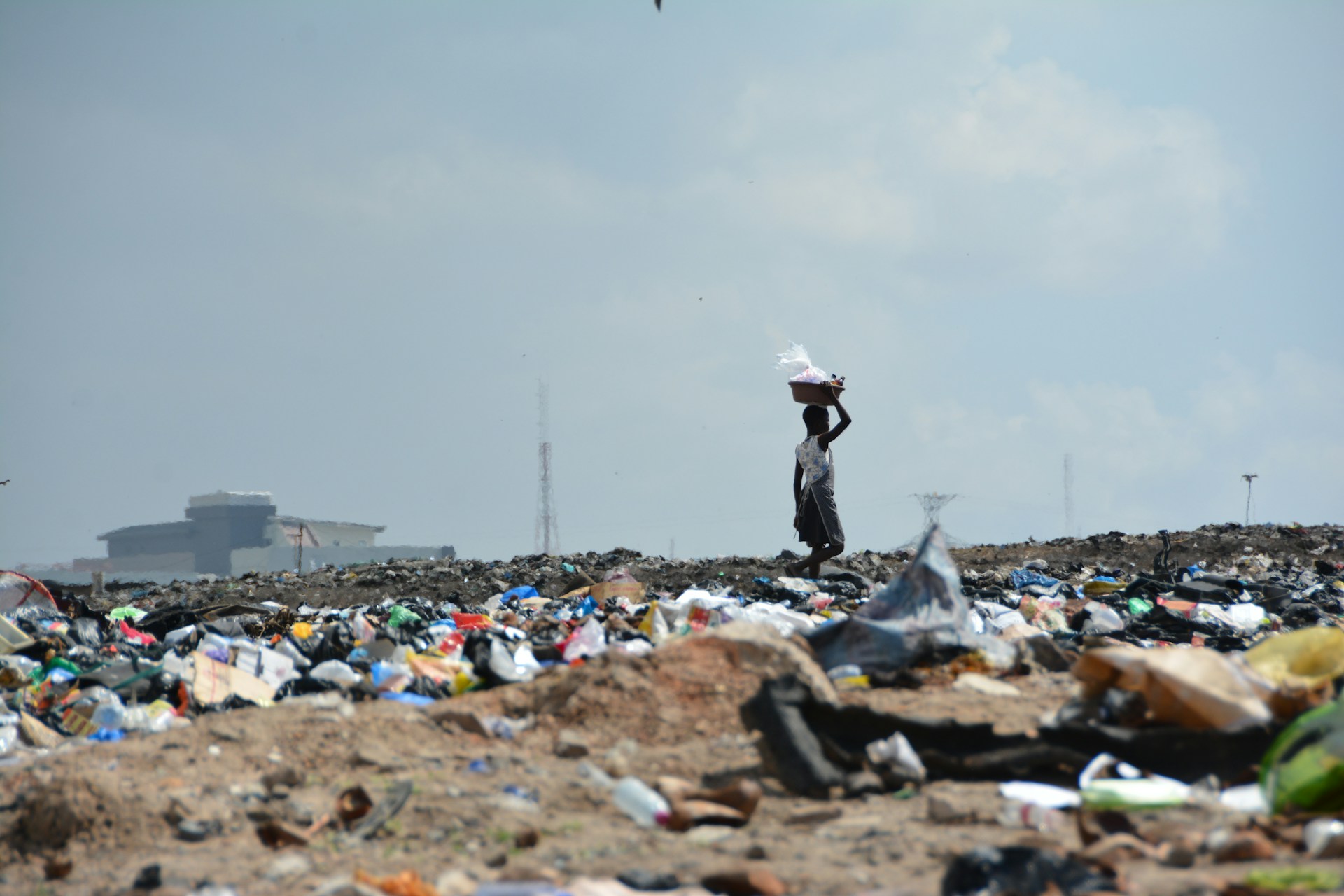
The future of plastics
Another fundamental and daunting conundrum confronting researchers today: As they, policymakers and the public seek plastic pollution solutions, the petrochemical industry continues churning out old and new plastics in astronomical amounts. Without action and research funding, the plastics crisis seems fated to outpace all attempts at solving it.
The world’s best hope to stem the flow of petrochemical pollution may be United Nations negotiations now underway to create a legally binding international agreement to address plastic pollution, with a final text due to be delivered at the end of this year.
But while the 65 nations of the High Ambition Coalition to End Plastic Pollution want a treaty that places binding caps on the production of new virgin plastics, along with a ban on the most toxic plastic chemicals, low-ambition nations want a voluntary agreement focused on recycling, with no production limits. Among the resisters to date are the United States, China, Russia, Iran and other oil- or plastics-producing nations. The plastics and petrochemical industry is also strongly opposed to regulation, sending nearly 200 lobbyists to the April 2024 U.N. treaty meeting in Ottawa, Canada.
If the result of the negotiations is a strong comprehensive treaty, then safe, scalable and economical solutions to the global plastics crises, like those described here, could become an essential part of the new circular plastics economy.
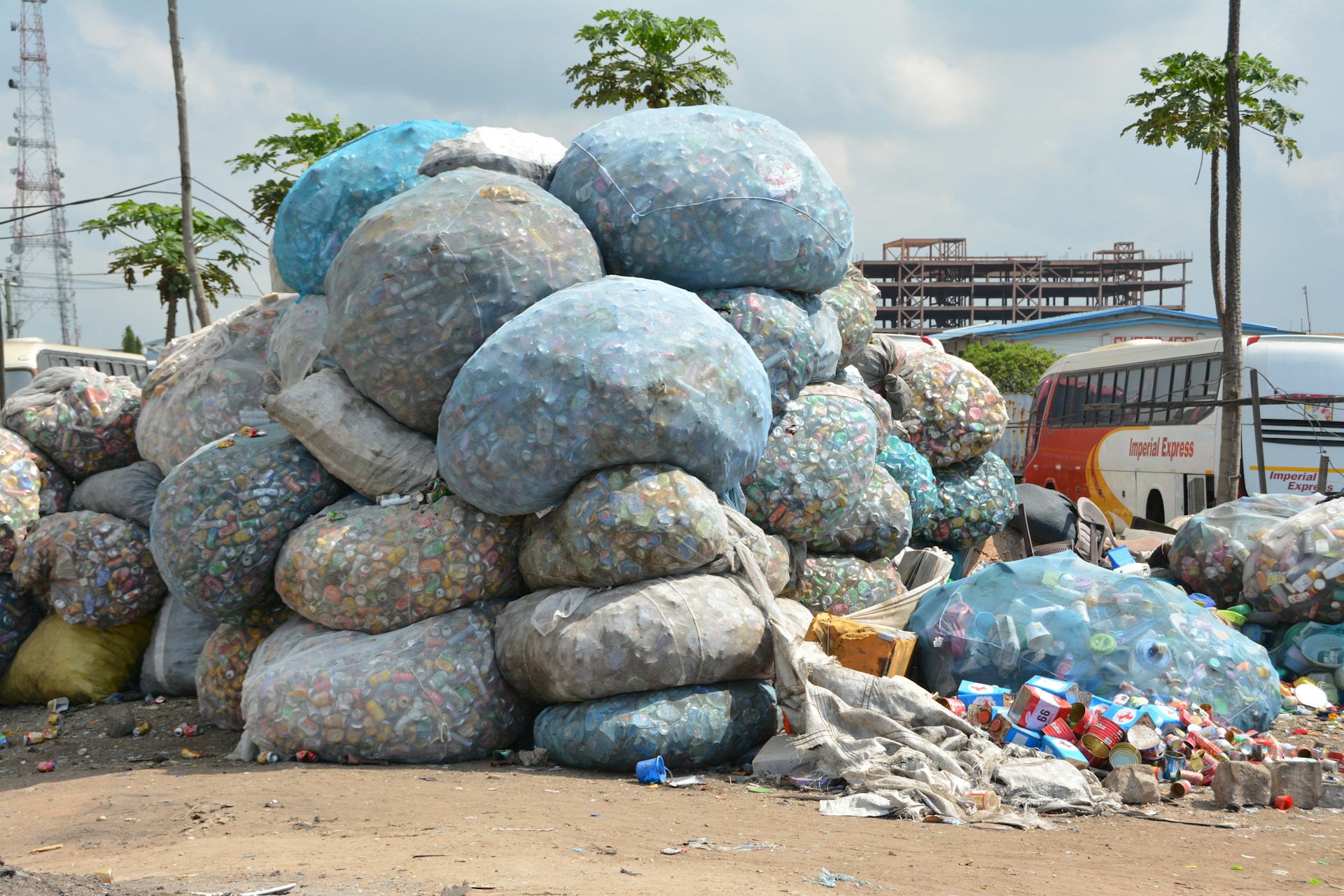
Nature’s promise in tackling pervasive pollutants
Plastics aren’t the only pollutants for which scientists are seeking nature-based solutions. Humans have created more than 350,000 novel chemicals, many of which are known to be toxic, with many more never tested, and all of which are entering the environment and potentially doing harm to biodiversity and human health.
So serious is this threat to life as we know it that researchers have recognized the introduction of novel entities into Earth’s ecosystems as a planetary boundary — a guardrail that scientists declared dangerously transgressed in January 2022.
Just a few nonplastic examples: per- and polyfluoroalkyl substances, known as PFAS or “forever chemicals,” are a group of more than 5,000 chemicals that are now ubiquitous environmental pollutants, and whose chemical structure makes them almost impossible to degrade. However, U.S. researchers have developed a cellulose filter that can absorb PFAS from contaminated groundwater. Once captured, microbes could help break down these persistent chemicals. Microbes isolated from sewage treatment plants have been identified that can break the strong chemical bonds in pollutants like PFAS.
Guo has also developed a polyphenol-based membrane that can capture radioactive uranium from wastewater, which has gained interest from the China National Nuclear Corporation. And researchers in Brazil and Malaysia are exploring the use of banana peels to absorb heavy metals such as lead, copper and iron from polluted water.
But if we hope to make nature-inspired technologies effective in combating human-caused pollution, we must learn from history. First, novel pollution solutions must be safety-tested thoroughly before they are implemented at scale. Second, humanity must avoid the temptation to use these new technologies as an excuse to continue down our current reckless path. Stricter rules are urgently needed now for the development and testing of novel chemicals. Applying the precautionary principle is key; otherwise, nature-based solutions will always be playing catch-up.
Banner image: Since 1950, humanity has produced more than 8.3 billion metric tons of plastic. Most has ended up in landfills or the environment, where it has harmful effects on wildlife, ecosystem functioning and human health. Nature-based solutions could help clean up plastic waste and put it to good use. Image by Wolfram Burner via Flickr (CC BY-NC 2.0).
At its fourth summit, 170 nations strive toward a global plastics treaty by 2025
Citations:
Wang, Y., Wang, M., Wang, Q., Wang, T., Zhou, Z., Mehling, M., … Guo, J. (2023). Flowthrough capture of microplastics through polyphenol‐mediated interfacial interactions on wood sawdust. Advanced Materials, 35(36). doi:10.1002/adma.202301531
Leppänen, I., Lappalainen, T., Lohtander, T., Jonkergouw, C., Arola, S., & Tammelin, T. (2022). Capturing colloidal nano- and microplastics with plant-based nanocellulose networks. Nature Communications, 13(1). doi:10.1038/s41467-022-29446-7
Liu, S. Y., Leung, M. M., Fang, J. K., & Chua, S. L. (2021). Engineering a microbial ‘trap and release’ mechanism for microplastics removal. Chemical Engineering Journal, 404, 127079. doi:10.1016/j.cej.2020.127079
Geyer, R., Jambeck, J. R., & Law, K. L. (2017). Production, use, and fate of all plastics ever made. Science Advances, 3(7). doi:10.1126/sciadv.1700782
Yoshida, S., Hiraga, K., Takehana, T., Taniguchi, I., Yamaji, H., Maeda, Y., … Oda, K. (2016). A bacterium that degrades and assimilates poly(ethylene terephthalate). Science, 351(6278), 1196-1199. doi:10.1126/science.aad6359
Tournier, V., Topham, C. M., Gilles, A., David, B., Folgoas, C., Moya-Leclair, E., … Marty, A. (2020). An engineered PET depolymerase to break down and recycle plastic bottles. Nature, 580(7802), 216-219. doi:10.1038/s41586-020-2149-4
Bombelli, P., Howe, C. J., & Bertocchini, F. (2017). Polyethylene bio-degradation by caterpillars of the wax moth Galleria mellonella. Current Biology, 27(8), R292-R293. doi:10.1016/j.cub.2017.02.060
Sanluis-Verdes, A., Colomer-Vidal, P., Rodríguez-Ventura, F., Bello-Villarino, M., Spinola-Amilibia, M., Ruiz-López, E., … Bertocchini, F. (2022). Wax worm saliva and the enzymes therein are the key to polyethylene degradation by Galleria mellonella. doi:10.1101/2022.04.08.487620
Sadler, J. C., & Wallace, S. (2021). Microbial synthesis of vanillin from waste poly(ethylene terephthalate). Green Chemistry, 23(13), 4665-4672. doi:10.1039/d1gc00931a
Bao, T., Qian, Y., Xin, Y., Collins, J. J., & Lu, T. (2023). Engineering microbial division of labor for plastic upcycling. Nature Communications, 14(1). doi:10.1038/s41467-023-40777-x
Sullivan, K. P., Werner, A. Z., Ramirez, K. J., Ellis, L. D., Bussard, J. R., Black, B. A., … Beckham, G. T. (2022). Mixed plastics waste valorization through tandem chemical oxidation and biological funneling. Science, 378(6616), 207-211. doi:10.1126/science.abo4626
Li, J., Li, X., Da, Y., Yu, J., Long, B., Zhang, P., … Dai, S. Y. (2022). Sustainable environmental remediation via biomimetic multifunctional lignocellulosic nano-framework. Nature Communications, 13(1). doi:10.1038/s41467-022-31881-5
Jin, B., Liu, H., Che, S., Gao, J., Yu, Y., Liu, J., & Men, Y. (2023). Substantial defluorination of polychlorofluorocarboxylic acids triggered by anaerobic microbial hydrolytic dechlorination. Nature Water, 1(5), 451-461. doi:10.1038/s44221-023-00077-6
Luo, W., Xiao, G., Tian, F., Richardson, J. J., Wang, Y., Zhou, J., … Shi, B. (2019). Engineering robust metal–phenolic network membranes for uranium extraction from seawater. Energy & Environmental Science, 12(2), 607-614. doi:10.1039/c8ee01438h
Castro, R. S., Caetano, L., Ferreira, G., Padilha, P. M., Saeki, M. J., Zara, L. F., … Castro, G. R. (2011). Banana peel applied to the solid phase extraction of copper and lead from river water: Preconcentration of metal ions with a fruit waste. Industrial & Engineering Chemistry Research, 50(6), 3446-3451. doi:10.1021/ie101499e
Mohamed, R. M., Hashim, N., Abdullah, S., Abdullah, N., Mohamed, A., Asshaary Daud, M. A., & Aidil Muzakkar, K. F. (2020). Adsorption of heavy metals on banana peel Bioadsorbent. Journal of Physics: Conference Series, 1532(1), 012014. doi:10.1088/1742-6596/1532/1/012014
FEEDBACK: Use this form to send a message to the author of this post. If you want to post a public comment, you can do that at the bottom of the page.